08/12/2015 - CTA Prototype Telescope Achieves "First Light"
|
|
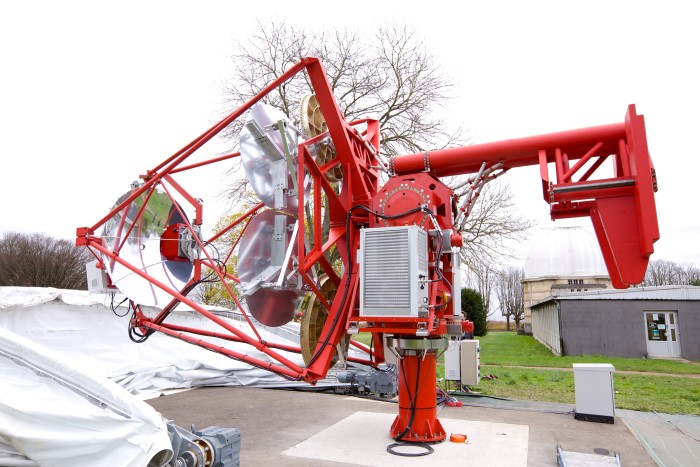
|
|
Left: GCT camera; right: GCT prototype on site the Observatoire de Paris, Meudon, France.
(credit: Akira Okumura)
|
On 2015, November 26, a prototype telescope proposed for the Cherenkov Telescope Array, the Gamma-ray Cherenkov Telescope (GCT), recorded CTA’s first ever Cherenkov light while undergoing testing at l’Observatoire de Paris in Meudon, France. The GCT is proposed as one of CTA’s small size telescopes (SSTs), covering the high end of the CTA energy range, between about 1 and 300 TeV (tera-electronvolts). Another SST prototype, the ASTRI telescope, captured the first optical image in May 2015 with its diagnostic camera.
In the two weeks leading up to the GCT prototype inauguration event on December 1, the GCT team battled poor weather to install and begin testing the GCT camera, left picture on top. On the evening of November 26, they turned the telescope away from a nearly full moon and the bright lights of Paris towards a clear patch of sky. After 20 seconds, a single event triggered the camera, then another – in just over 300 seconds 12 events were captured. These triggers could have been caused by fluctuations in the bright night sky, but it was instantly clear that they were, in fact, what the team was looking for – images of air showers created in the atmosphere by cosmic rays.
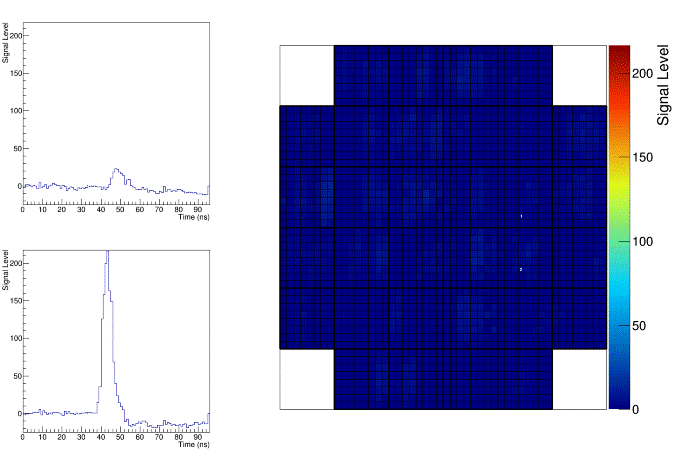
Image of an event captured by the GCT
(credit: CTA Collaboration)
The image shown below is one of the events captured by the team. It shows the maximum amount of light captured in each of the camera’s 2048 pixels over 100 frames. CTA astronomers will use images like this to determine the incoming direction and energy of the particle that created the air shower.
"With the tough weather conditions, we only had about an hour-long window to gather as much data as we could," said GCT Camera Coordinator Dr. Richard White. "We look forward to clearer, darker skies so we can test the camera’s performance in more ideal conditions."
"This is a major milestone for the GCT and we hope for CTA." said GCT Spokesperson Prof. Tim Greenshaw. "Our design for the CTA telescopes that will detect the highest energy light hitting the earth’s atmosphere from space has been proven to work; we are one step closer to developing a deeper understanding of where and how that light is produced."
Hélène Sol, Research Director at Centre National de la Recherche Scientifique (CNRS) and GCT Deputy Spokesperson added: "I would like to congratulate all the GCT team who have made this possible, especially the group who worked day and night over the last couple of weeks to get these pictures."
In order to detect the short flashes of light produced by cosmic rays and gamma rays as they hit the earth’s atmosphere, the telescope's camera has to be about a million times faster than a DSLR camera. To do this, it uses high-speed digitisation and triggering technology capable of recording images at a rate of one billion frames per second and sensitive enough to resolve single photons.
These first pictures are just the beginning for the GCT. The prototype telescope and camera will undergo rigorous testing over the next year, then the team intends to build 35 cameras and telescopes for the CTA Observatory based on the results of the testing process.
"We’re extremely pleased with the progress and performance of the GCT prototype and all of the CTA prototypes," said CTA Project Manager Christopher Townsley. "We look forward to seeing the results of further testing as we near the construction phase of the project."
CTA
CTA is a global initiative to build the world’s largest and most sensitive high-energy gamma-ray
observatory. Over 1,000 scientists and engineers from 32 countries and over 170
research institutes participate in the CTA project. CTA will serve as an open observatory to a
wide physics and astrophysics community and provide a deep insight into the non-thermal,
turbulent, high-energy universe. The CTA observatory will detect high-energy radiation with
unprecedented accuracy and approximately 10 times the sensitivity of current instruments,
providing novel insights into some of the most extreme and violent events in the universe.
At least three telescope types are required to cover the full CTA energy range. The sensitivity
in the core energy range between 100 GeV and 10 TeV will be dominated by up to 40
Medium-Size Telescopes (MSTs) distributed over both array sites in the northern and
southern hemispheres. Four Large-Size Telescopes (LSTs) and around 70 SSTs will be
essential to extend the energy range below 100 GeV and above a few TeV. The GCT adds to
the current complement of CTA prototypes located around the world: the SST-1M (Krakow,
Poland), SST-2M ASTRI (Serra la Nave, Italy), MST (Zeuthen, Germany) and the LST (La
Palma, Spain).
|
Press release: CTA
08/12/2015 - The construction of KM3NeT, the next generation neutrino telescope, has begun
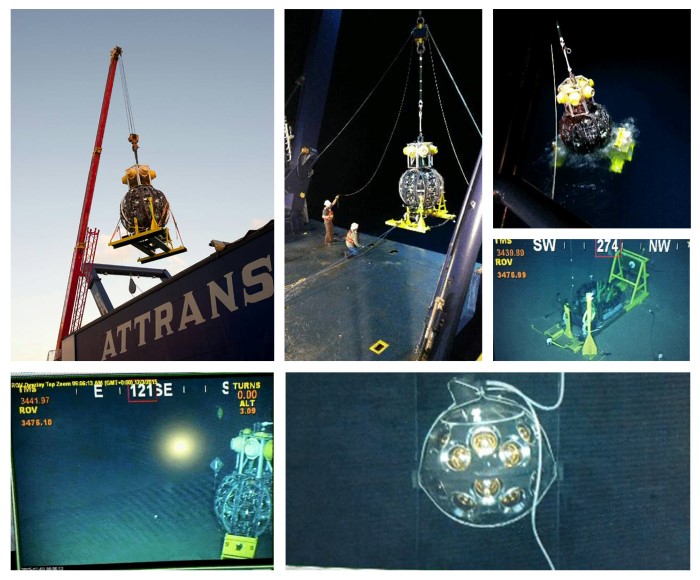
The neutrino detection string wound around the spherical deployment frame prior to
deployment on board the boat to touch down of the deployment frame
on the sea floor
(underwater pictures: Display screens in control room).
(credit: KM3Net Collaboration)
In the early morning of 2015 December 3, scientists and engineers started the installation of
KM3NeT, which once completed, will be largest detector of neutrinos in the Northern
Hemisphere. Located in the depths of the Mediterranean Sea, the infrastructure will be used
to study the fundamental properties of neutrinos and map the high-energy cosmic neutrinos
emanating from extreme cataclysmic events in the Universe.
On board the Ambrosius Tide deployment boat, the first string – wound, like a ball of wool, around a spherical frame – arrived at the location of the KM3NeT-Italy site south of Sicily. It was anchored to the seabed at a depth of 3,500 m and connected to a junction box, already present on the seafloor, using a remotely operated submersible controlled from the boat. The junction box is connected by a 100 km cable to the shore station located in Portopalo di Capo Passero in the south of Sicily.
Marco Circella, the technical coordinator of KM3NeT explains, "The large depth of sea water shields the telescope from particles created by cosmic rays in the atmosphere above the telescope. Constructing such a large infrastructure at these depths is a tremendous technical challenge. Making the underwater connections requires custom-designed electrical and fibre optic connectors. The crew of the Ambrosius Tide are experts in performing such delicate submarine operations."
After verification of the quality of the power and fibre optic connections to the shore station, the go ahead was given to trigger the unfurling of the string to its full 700 m height. During this process, the deployment frame is released from its anchor and floats towards the surface while slowly rotating. In doing so, the string unwinds from the spherical frame, eventually leaving behind a vertical string. The string was then powered on from the shore station and the first data from the sensor modules started streaming to shore.
The successful acquisition of data from the abyss with the novel technology developed by the KM3NeT Collaboration is a major milestone for the project. It represents the culmination of more than ten years of research and development by the many research institutes comprising the international Collaboration.
Maarten de Jong, spokesperson and director of KM3NeT, said: “This important step in the verification of the design and the technology will allow the KM3NeT Collaboration to proceed with confidence toward the mass production of detection strings and their installation at thesites in the Mediterranean Sea off-shore from Italy and France. A new era in neutrino astronomy has begun”
KM3Net
Neutrinos are the most elusive of elementary particles and their detection requires the instrumentation of enormous volumes: The KM3NeT neutrino telescope will occupy more than a cubic kilometre of seawater. It comprises a network of several hundred vertical detection strings, anchored to the seabed and kept taut by a submerged buoy. Each string hosts 18 light sensor modules equally spaced along its length. In the darkness of the abyss, the sensor modules register the faint flashes of Cherenkov light that signal the interaction of neutrinos with the seawater surrounding the telescope.
The international KM3Net Collaboration consists of 35 research groups from France, Germany, Greece, Italy, Morocco, The Netherlands, Poland and Spain.
|
Press release: KM3Net
02/12/2015 - A search for cosmic-ray sources with IceCube, the Pierre Auger Observatory, and the Telescope Array
High-energy neutrinos are thought to be excellent cosmic messengers when exploring the extreme universe: they don’t bend in magnetic fields as cosmic rays (CRs) do and they are not absorbed by the radiation background as gamma rays are. However, it turns out that the deviation of some CRs, namely protons, is expected to be only a few degrees at energies above 50 EeV. This opens the possibility for investigating common origins of high-energy neutrinos and CRs.
In a new study by the IceCube, Pierre Auger, and Telescope Array Collaborations, scientists have looked for correlations between the highest energy neutrino candidates in IceCube and the highest energy CRs in these two cosmic-ray observatories. The results, submitted today to the Journal of Cosmology and Astroparticle Physics, have not found any correlation at discovery level. However, potentially interesting results have been found and will continue to be studied in future joint analyses.
|
|
|
|
Maps in Equatorial and Galactic coordinates showing the arrival directions of the IceCube cascades (black dots) and tracks (diamonds), as well as those of the UHECRs detected by the Pierre Auger Observatory (magenta stars) and Telescope Array (orange stars). The circles around the showers indicate angular errors. The black diamonds are the HESE tracks while the blue diamonds stand for the tracks from the through-going muon sample. The blue curve indicates the Super-Galactic plane. Image: IceCube, Pierre Auger and Telescope Array Collaborations.
(credit: IceCube, Pierre Auger, TA collaborations)
|
|
The IceCube astrophysical neutrino flux is consistent with an isotropic distribution, which suggests that most neutrinos have an extragalactic origin. The intensity of this flux is also found to be close to the so-called Waxman-Bahcall flux, which is the rate assuming that ultra-high-energy CRs (UHECRs) are mainly protons and have a power-law
spectrum. In this scenario, primary cosmic rays collide to a significant extent with photons and neutrons within the source environment, resulting in mainly protons escaping from these sources.
The UHECRs detected by the Pierre Auger Observatory (Auger) and the Telescope Array (TA) that were used in this study have energies above 50 EeV, since at the highest energies cosmic rays are deflected the least. UHECRs produce neutrinos that carry only 3-5% of the original proton energy, i.e., neutrinos that would have energies of at least several hundred PeVs for the CR sample of this work. However, we expect that the sources of these UHECRs will also produce lower energy CRs, which would then produce neutrinos in the energy range—30 TeV to 2 PeV—observed in IceCube. And this is the idea behind this search: to look for a statistical excess of neutrinos in IceCube from the direction of cosmic rays in the Auger and TA and, thus, their sources.
Not a simple search, but definitely worth trying to study since searches for the most obvious potential CR sources using IceCube neutrinos have not been successful so far. The major challenges of this search are: i) CRs do not precisely point to their sources, and our knowledge of the deviations produced by the galactic magnetic fields is limited; ii) cascade neutrino events—mainly produced by electron and tau neutrinos—in IceCube are characterized by large angular uncertainties; and iii) IceCube neutrino candidates include background muon events due to the interaction of CRs with the Earth’s atmosphere.
Researchers have used three different analyses to tackle these challenges. They first searched for cross-correlations between the number of CR-neutrino pairs at different angular windows and compared them to expectations for the null hypothesis of an isotropic UHECR flux. Then, they used a stacking likelihood analysis that looked for the combined contribution from different sources. These two searches used both cascade and track neutrino events from the astrophysical neutrino fluxes measured in IceCube (HESE and high-energy throughgoing muons). IceCube track neutrino signatures are produced by charged-current interactions of muon neutrinos and have an angular uncertainty of less than one degree. Finally, they performed a third study, a stacking search using the neutrino sample used for the four-year point-source search in IceCube, which includes track neutrino candidates only.
The results obtained are all below 3.3 sigma. There is a potentially interesting finding in the analyses performed with the set of high-energy cascades. When compared to an isotropic flux of neutrinos (fixing the positions of the cosmic rays) to consider the effect of anisotropies in the arrival directions of cosmic rays, the significance is 2.4 sigma for the cross-correlation analysis. These results were obtained with relatively few events and the collaborations will update the analyses in the future with additional statistics to follow their evolution.
Press release: IceCube, Pierre Auger Observatory
Publication: Search for correlations between the arrival directions of IceCube neutrino events and ultrahigh-energy cosmic rays detected by the Pierre Auger Observatory and the Telescope Array, IceCube, Pierre Auger and Telescope Array Collaborations: M.G.Aartsen et al., submitted to Journal of Cosmology and Astroparticle Physics (arXiv:1511.09408)
16/11/2015 - AugerPrime Symposium

Celebrating 15 years of achievements and
signature ceremony of a new International Agreement for the next 10 years
The Pierre Auger Observatory is the world’s leading science project for the exploration of cosmic rays. More than 500 scientists from 16 countries have been working together since 1998 in the Province of Mendoza, Argentina, to elucidate the origin and properties of the most energetic particles in the Universe, coming to us from the far reaches of the cosmos. The Pierre Auger Observatory measures gigantic showers of relativistic particles that are the result of collisions between the very rare, highest-energy cosmic rays and atomic nuclei of the atmosphere. Properties of such air showers are used to infer the energy, direction, and mass of the cosmic particles.
Results from the Pierre Auger Observatory have brought new fundamental insights into the origin and nature of highest-energy cosmic rays. One of the most exciting results is the experimental proof that at the highest energies (7 orders of magnitude above what can be achieved at CERN’s Large Hadron Collider) the cosmic-ray flux decreases much faster than at low energies. Data indicate that this flux suppression marks the limiting energy of the most powerful cosmic particle accelerators. An even more detailed measurement of the nature of cosmic particles at the highest energies is crucial to understand the mechanisms responsible for this decrease, and to identify the astrophysical sites violent enough to accelerate particles to such tremendous energies.
The AugerPrime upgrade to the Observatory enhances the 1660 existing surface detectors (water tanks sensitive to Cherenkov light generated by the shower products) with new scintillation detectors, so that electromagnetic and muonic shower particles can be separated more efficiently. This in turn, together with smaller area of buried muon detectors, improves the determination of the mass of the primary cosmic rays, otherwise not directly measurable. Faster and more powerful electronics also facilitates the readout of the new detector components and enhances the overall performance of the Observatory elements.
A symposium, held on November 15-16, 2015 gathers collaborators and science funding agency representatives for the signing of a new international agreement for continued operation of the Pierre Auger Observatory until 2025. This will provide the basis for doubling the present statistics with the upgraded Observatory, and for solving the long-standing puzzle of the origin of the most energetic particles in the Universe.
|
 |
 |
|
A prototype station of AugerPrime in the Argentinian Pampa: The Water Cherenkov Detector containing 12000 liters of water (bottom) is augmented by a 4 square meter scintillation detector (top) to enhance the particle identification capabilities. |
Headquarter of the Pierre Auger Observatory in Malargüe, Province of Mendoza, in Argentina. |
|
 |
 |
|
Group picture of the participants of the AugerPrime Symposium. |
AugerPrime Symposium. |
|
 |
|
|
AugerPrime Symposium. From left to right: Prof. Karl Heinz Kampert, spokesperon of the Pierre Auger Observatory, Bergische Universität Wuppertal, Germany; Alberto Lamagna, Gerente de Área de Investigación y Aplicaciones No Nucleares de la Comisión Nacional de Energía Atómica (CNEA), Argentina; Dr. Lino Barañao, Ministro de Ciencia, Tecnología e Innovación Productiva; Juan Antonio Agulles, Intendente del Departamento de Malargüe, Argentina; Prof. Fernando Ferroni, Presidente of the Instituto Nazionale di Fisica Nucleare, Italy; Ing. Julio Cobos, Senador Nacional Electo por la Provincia de Mendoza, Argentina; Ing. Rolando Baldasso, Ministro de Infraestructura de la Provincia de Mendoza. |
Signature ceremony. Were present from left to right: Prof. Mario-Pimenta, LIP, Portugal; Dr. Lino Barañao, Ministro de Ciencia, Tecnología e Innovación Productiva; Dr. Carola Dobrigkeit, UNICAMP, Brazil; Prof. Fernando Ferroni, INFN, Italy; Prof. Johannes Bluemer, KIT, Karlsruhe, Germany; Jan Ridky, ELI, Czech Republik; Alberto Lamagna, CNEA, Argentina; Dr. Reynald Pain, CNRS - IN2P3, Paris, France; Prof. Stan Bentvelsen, Nikhef, Netherlands; Ing. Rolando Baldasso, Ministro de Infraestructura de la Provincia de Mendoza. |
Material: Pictures above (high-res, web-HD), individual pictures of signatory (high-res, web-HD).
Press release: Pierre Auger Observatory
11/11/2015 - XENON1T inauguration at Gran Sasso
.JPG)
XENON1T inauguration
(credit: INFN-LNGS)
On November 11, an international collaboration of scientists inaugurated the new XENON1T instrument designed to search for dark matter with unprecedented sensitivity, at the Gran Sasso Underground Laboratory of INFN in Italy.
The event took place at the Gran Sasso National Laboratory of the Italian National Institute for Nuclear Physics (INFN-LNGS), the largest underground laboratory in the world for astroparticle physics.
The inauguration was attended by the XENON scientists along with guests from funding agencies as well as journalists and colleagues. About 80 visitors were able to join the ceremony directly at the experimental site in the 100 m-long, 20 m-wide and 18 m-high hall B of LNGS, which is itself below 1 400m of rock. Here, the new XENON1T instrument is installed inside a 10 m-diameter water tank to shield it from radiation which originates from the environment. Even more guests followed the introductory presentations in the LNGS auditorium, where Elena Aprile, Professor at Columbia University (New York) and founder of the XENON project, illustrated the evolution of the XENON program from the early beginnings with a 3 kg detector 15 years ago to the present-day instrument XENON1T with a total mass of 3 500kg.
Once fully operational, XENON1T will be the most sensitive dark matter experiment in the world. The detector installation has been completed just a few days ago and the first tests of its performance have already been started. The first science results are expected early 2016, as only one week of good data is sufficient to yet again take the lead in the field. The design goal of the experiment will be reached after two years of data taking, as the collaboration explains in a detailed sensitivity study published at the same time as the inauguration. The ultimate goal is the detection of the dark matter particle. Still, even if there are only some hints found after two years of operation, the XENON collaboration will be in an excellent position to move forward, as the next phase of the project, XENONnT, is already being prepared. It will largely use already existing infrastructure, and will increase the sensitivity to dark matter by another order of magnitude
Dark Matter
Dark matter is one of the basic ingredients of the Universe, and efforts to detect it with laboratory-based experiments have been ongoing for decades. However, until today dark matter has been observed only indirectly via its gravitational interactions – the interactions that govern the dynamics of the Cosmos at all length-scales. It is expected that dark matter is made of a new, stable elementary particle which has so far escaped detection. About 100 000 dark matter particles are expected to pass through an area of 1 cm² per second. The fact that these particles have not yet been directly detected puts stringent constraints on their tiny interaction probability with the atoms of ordinary matter. It also implies that more sensitive instruments are required to find the rare signature of the dark matter particle.
|
XENON1T
XENON1T employs the noble gas xenon as the dark matter detection material, which must be made ultra-pure and cooled down to –95°C to make it liquid. The large-mass instrument features an extremely low radioactive background in order to be able to identify the rare events from dark matter interactions. For this reason, the XENON scientists have carefully selected all materials used in the construction of the detector, ensuring that their intrinsic contamination with radioactive isotopes meets the experiment's low-background strict requirements.
The XENON1T detector measures the tiny flashes of light and charge which are generated when a particle interacts with the xenon. The scientists use this information to reconstruct the position of the particle interaction within the detector, as well as the deposited energy and whether the interaction may have been induced by dark matter. The light is observed by 248 sensitive photosensors, which are each capable of detecting even single photons. A vacuum-insulated double-wall cryostat, essentially a gigantic version of a thermos flask, contains the cryogenic xenon and the dark matter detector. The xenon gas is cooled down and purified in the three-story tall XENON building, a fancy installation with a transparent glass facade right next to the water tank, allowing visitors to actually see what the scientists are doing inside. A gigantic stainless-steel sphere equipped with pipes and valves is installed on the ground floor. It can accommodate 7.6 tons of xenon in liquid and gaseous form, more than two times the capacity needed for XENON1T. This will allow the collaboration to swiftly increase the sensitivity of the experiment by using a larger mass detector in the near future.
The international XENON Collaboration, consisting of 21 research groups from the United States, Germany, Italy, Switzerland, Portugal, France, the Netherlands, Israel, Sweden and United Arab Emirates, celebrated the inauguration of their new XENON1T instrument today, which will search for dark matter with unprecedented sensitivity.
|
Press release: INFN
10/11/2015 - Breakthrough Prize in Fundamental Physics for Neutrino Physicists
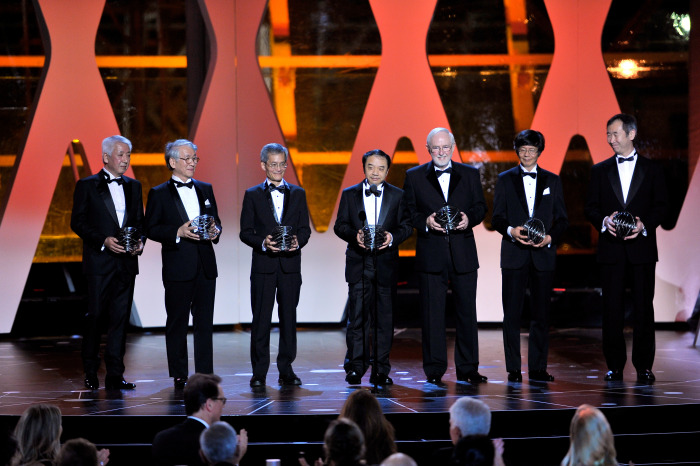
Breakthrough winners onstage during the 2016 Breakthrough Prize Ceremony
on November 8, 2015 in Mountain View, California.
(Photo by Steve Jennings/Getty Images for Breakthrough Prize)
(Copyright Getty Images 2015)
Scientists from five experiments have been awarded together the 2016 Breakthrough Prize in Fundamental Physics. These five collaborations made groundbreaking contributions to the discovery and exploration of neutrino oscillations, which leads to important insight into the structure of the universe. Among the award winners is also the Dresden physicists Kai Zuber. He shares the prize with a total of 1 376 colleagues who, like him, work on one of the five large-scale experiments: Daya Bay (China), KamLAND (Japan), K2K / T2K (Japan), Sudbury Neutrino Observatory SNO (Kanada) und Super-Kamiokande (Japan). The Breakthrough Prize is endowed with a total of 3 million US dollars and is awarded by a foundation that was founded by the Russian entrepreneur Yuri Milner.
Mirroring the choice of the Nobel committee earlier this year, the prize has awarded neutrino physicists Takaaki Kajita and Arthur B. McDonald, spokesperson respectively of the Super-Kamiokande detector and the SNO experiment. With the Breakthrough Prize all scientists who were involved in these experiments have now been awarded. This includes Kai Zuber, a professor of nuclear physics at the TU Dresden, who is working within SNO since 15 years.
Neutrinos are elementary particles which come in three flavors. These flavors can be converted into one another, which is described by the term neutrino oscillations. This process has been studied and proven in the awarded experiments. In addition, these results imply the idea that neutrinos are not massless. Dresdner nuclear physicists with Kai Zuber are now working on follow-up experiment of SNO, called SNO +. In addition, Zuber and his group are involved into other neutrino experiments such as GERDA or COBRA. The investigations have among other objectives, to determine the exact values of the neutrino masses.
Press release: TU Dresden
06/10/2015 - 2015 Nobel Prize in Physics for Astroparticle Physics
The Royal Swedish Academy of Sciences has decided to award the Nobel Prize in Physics for 2015 to Takaaki Kajita (University of Tokyo) and Arthur B. McDonald (Queen’s University) "for the discovery of neutrino oscillations, which shows that neutrinos have mass".
The discovery rewarded with the 2015 Nobel Prize in Physics have yielded crucial insights into the all but hidden world of neutrinos. After photons, neutrinos are presumably the most numerous in the entire cosmos. The Earth is constantly bombarded by them. Many neutrinos are created in reactions between cosmic radiation and the Earth’s atmosphere. Others are produced in nuclear reactions inside the Sun. Thousands of billions of neutrinos are streaming through our bodies each second. Hardly anything can stop them passing; neutrinos are nature’s most elusive known elementary particles.
The possibility of neutrino flavour change, i.e. neutrino oscillations, has been discussed already shortly after neutrinos were first discovered experimentally in 1956. However, it was around the turn of the millennium that two convincing discoveries validated the actual existence of neutrino oscillations.
In 1998, at Neutrino’98, the largest international neutrino conference series, Takaaki Kajita of the Super-Kamiokande Collaboration presented the first evidence that neutrinos from the atmosphere switch between two identities on their way to the Super-Kamiokande detector in Japan. Also miraculous had been the measured flux of electron-type neutrinos from the Sun which appeared to be only about 1/3 of the theoretical expectation.
In addition, in 2001/2002, the Sudbury Neutrino Observatory (SNO) Collaboration, led by Arthur B. McDonald, were able to measure both, the electron-type neutrino flux and the total neutrino flux of all flavours from the Sun. They confirmed a deficit in electron-type neutrinos – which lead to the 2002 Nobel Prize in Physics to Raymond Davis and Masatoshi Koshiba. In 2004 SNO published clear evidence for conversion of electron-type neutrinos from the Sun into muon-or tau-neutrinos by observing the expected neutrino flux from solar fusion reactions.
The discovery that neutrinos can convert from one flavour to another and therefore have non-zero masses is a major milestone for elementary particle physics and astroparticle physics. It represents compelling experimental evidence for the incompleteness of the Standard Model – which requires neutrinos to be massless – as a description of nature.
Today, understanding the nature of the neutrino is of prime importance – not only for elementary particle physics but also for astrophysics and cosmology. The answer to several key questions about the nature of the neutrino may lead to the discovery of new theories beyond the Standard Model. What are the neutrinos’ masses? Why are they so light-weight? Are there more types than the three currently known? Are neutrinos their own antiparticles? Why are they so different from other elementary particles? Neutrino oscillations and the connected issues of the nature of the neutrino, neutrino masses and possible CP violation among leptons are today major research topics in particle and astroparticle physics.
The discovery that is awarded with this year’s Nobel Prize in Physics has yielded crucial insights into the almost entirely hidden world of the neutrino. The prize is received at a time where the investigations are continued and research of neutrino receives an unprecedented attention around the world. Several neutrino experiments are key projects of the Helmholtz Alliance for Astroparticle Physics and pursued by the participating scientists. New discoveries of the neutrino’s closely guarded secrets are expected and will continue to change our understanding of the history, structure and future fate of the universe.
2015 Nobel Prize Laureates
Takaaki Kajita was born in 1959 in Higashimatsuyama, Japan. He earned his PhD in 1986 from University of Tokyo, Japan. He is currently director of the Institute for Cosmic Ray Research and a professor at University of Tokyo in Kashiwa, Japan.
In 2013 Takaaki Kajita was laureate of the Julius Wess Award of KCETA at the Karlsruhe Institute of Technology (KIT).
Arthur B. McDonald was born in 1943 in Sydney, Canada. He earned his PhD in 1969 from California Institute of Technology in Pasadena, California. He is a professor emeritus at Queen’s University in Kingston, Canada.
|
Sudbury Neutrino Observatory
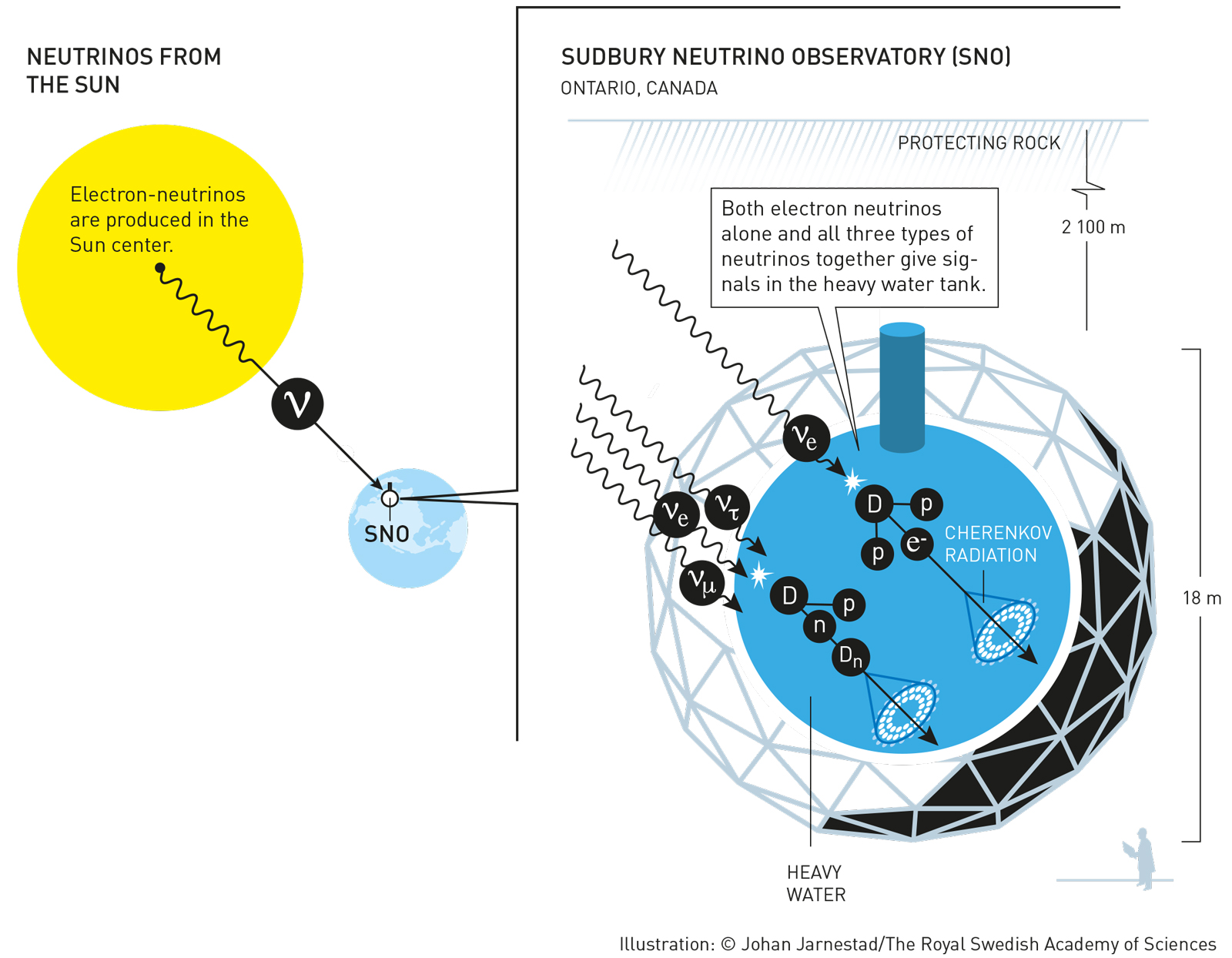
Sudbury Neutrino Observatory detects neutrinos from the Sun.
Sudbury Neutrino Observatory consists of 1,000 tons of heavy water more than 2,000 m below the surface. It detects neutrinos from the Sun, where only electron-neutrinos are produced. The reactions between neutrinos and the heavy water in the tank yielded the possibility to separately measure both electron-neutrinos alone and all three types of neutrinos combined. It was discovered that the electron-neutrinos were fewer than expected, while the total number of all three types of neutrinos combined matches the expectations. The conclusion is that some of the electron-neutrinos have changed into another identity.
Sudbury Neutrino Observatory is a cooperation of scientists from Canada, Germany, Portugal, UK and USA. Germany is represented with the group of Prof. Kai Zuber from the HAP partner Technische Universität Dresden.
|
Super-Kamiokande
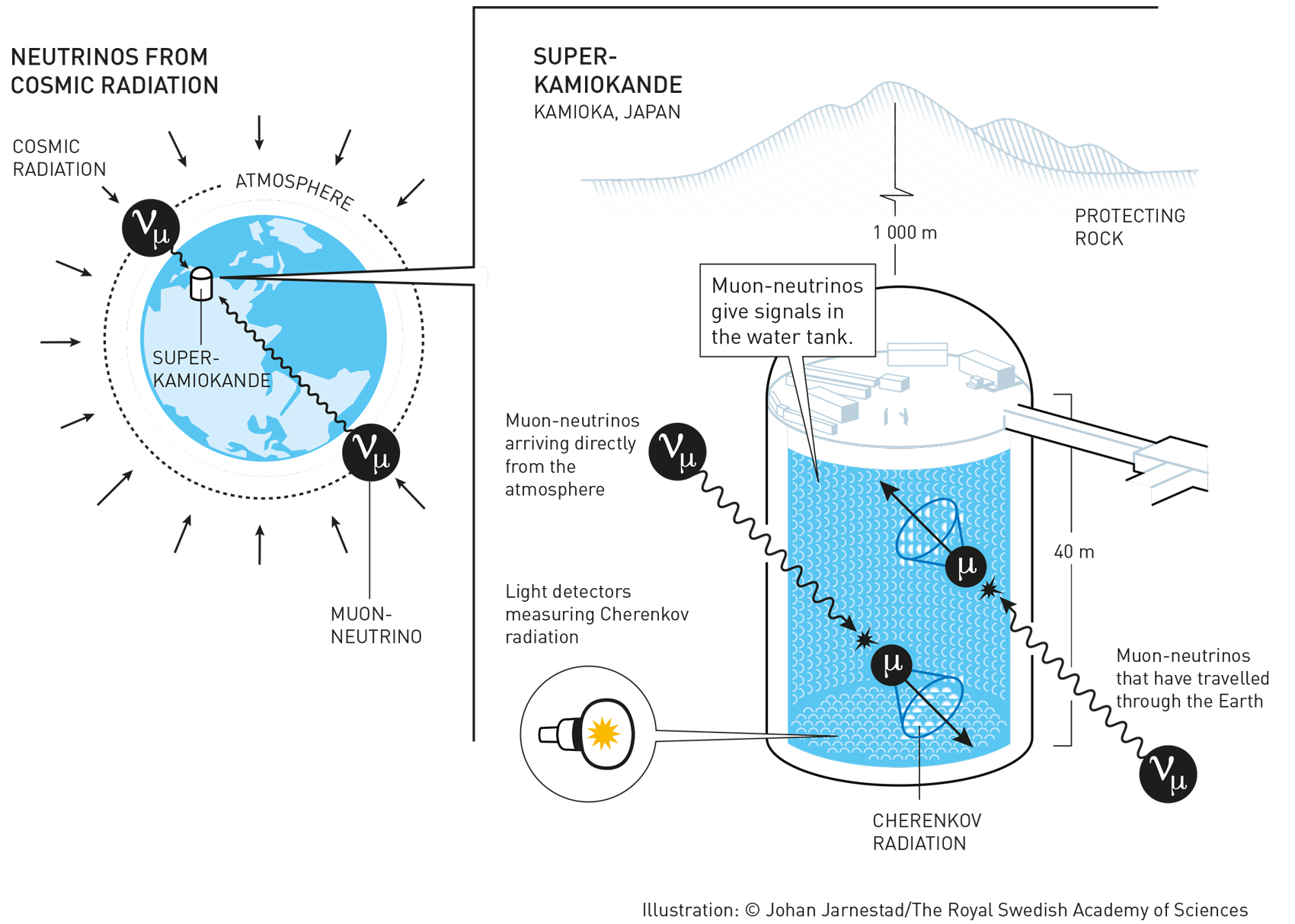
Super-Kamiokande, a detector built 1,000 metres below the Earth’s surface detecting atmospheric neutrinos.
Super-Kamiokande is a gigantic detector built 1,000 metres below the Earth’s surface. It consists of a tank, 40 metres high and as wide, filled with 50,000 tonnes of water. It detects atmospheric neutrinos. When a neutrino collides with a water molecule in the tank, a rapid, electrically charged particle is created. This generates Cherenkov radiation that is measured by the light sensors. The shape and intensity of the Cherenkov radiation reveals the type of neutrino that caused it and the direction from where it came. The muon-neutrinos that arrived at Super-Kamiokande from above were more numerous than those that travelled through the entire Earth. This indicated that the muon-neutrinos that travelled longer had time to change into another identity on their way.
Super-Kamiokande is a cooperation of scientists from Japan, Canada, Korea, Spain and USA.
|
30/09/2015 - Senior Fellows of the Helmholtz Alliance for Astroparticle Physics 2015
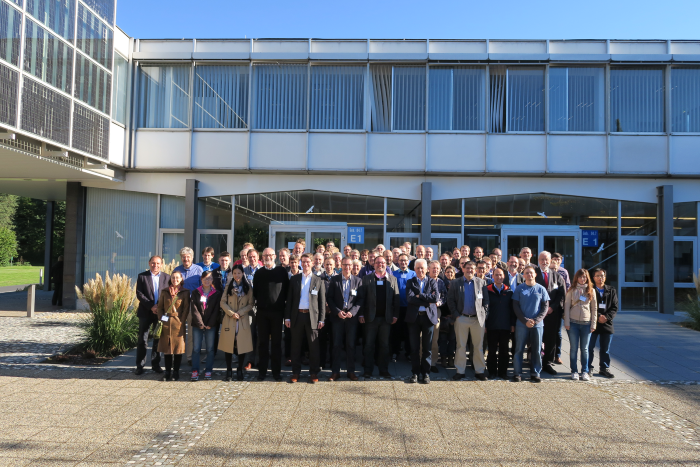
Group picture of the first annual meeting Matter and the Universe
(credit: HAP/A.Chantelauze)
On September 29-30 took place the annual meeting of the Helmholtz Programme Matter and the Universe at Forschungszentrum Jülich. This first annual meeting of the programme at the Research Center Jülich brought together the different programme communities with activities in elementary particle physics, hadron physics, nuclear physics and astroparticle physics in order to strengthen the co-operation across the different programme topics.
|
|
|
|
Left: Dr. Keilhauer (HAP spokesperson) and Prof. Dr. Grupen, Right: Dr. Keilhauer and Prof. Dr. Meyer
(credit: HAP/A.Chantelauze)
|
During the meeting also took place the Senior Fellow award ceremony of the Helmholtz Alliance for Astroparticle Physics. The title of Senior Fellow of the Helmholtz Alliance for Astroparticle Physics in 2015 has been awarded to:
- Prof. Dr. Claus Grupen (University of Siegen),
- Prof. Dr. Hinrich Meyer (University of Wuppertal).
The two retired scientists are awarded a Senior Fellowship, travel funds for conferences and schools associated with HAP as well as funding opportunities for scientific projects. Thus, the recognized scientists can continue to be involved in current research and to share their experience and knowledge within the Alliance.
Press release:
Uni. Siegen,
Uni. Wuppertal
30/09/2015 - First gamma-ray Images from new H.E.S.S. camera
The new camera, mounted at telescope CT1.
(credit: Stefan Klepser/DESY )
The first of four new gamma-ray cameras has recorded its first light at the gamma-ray observatory H.E.S.S. (High Energy Stereoscopic System) in Namibia. In July, a team of ten colleagues from DESY Zeuthen had upgraded the camera and installed 200 electronic boards and a new ventilation system. After the integration into the network and data acquisition system, first cosmic gamma-ray images could now be acquired.
With H.E.S.S. physicists look at some of the most energetic objects and processes in the universe, including supernova explosions, active galaxies with enormous black holes and fast rotating corpses of burnt-out giant suns. The cameras of the H.E.S.S. I telescopes are in operation since 2003. They increasingly suffer from ageing and the harsh environmental conditions in the Khomas highland. To allow for continuous stable operations in the coming years, a major hardware upgrade for these technologically sophisticated devices was developed in the past two years. The new components were tested in an identical copy of the camera, which was set up in Zeuthen.
"These tests in the lab were quite essential," concludes Stefan Klepser, project leader at DESY, "but they cannot fully mimic all possible problems that occur in the field. I'm very relieved that all installations could really be accomplished within the three weeks that we planned for." All together, 20 engineers and scientists took part in the installations on site, half of which were from DESY, and the other half were H.E.S.S. colleagues from Windhoek, Heidelberg, Paris and Berlin.
The new, faster camera can resolve and digitise the light flashes produced by gamma rays in the atmosphere, which only last few nanoseconds. "The design of the electronic boards was a big challenge. Many of the boards are composed of more than 1,000 single pieces," explains electronics designer Holger Leich. "Since we could produce those at DESY in Hamburg, we could ensure the high quality standards that we needed.
Still, the mere installation of hardware components is only half the way to a working camera. "The software for the new camera was all developed from scratch," says Gianluca Giavitto from Zeuthen, who led the integration of the new camera into the whole telescope array that took several weeks. "And since the whole camera works with Ethernet technologies, we have made use of very modern technologies, part of which are also used at Google or Facebook. This has simplified the development, and improved the stability of the system."
It will need more time to get to a complete calibration and analysis of the new system, but Christian Stegmann, head of DESY Zeuthen, is confident: "These images demonstrate the basic functionality of the camera, which means green light for the production of the remaining three, to be installed in 2016." His confidence is shared by collaborators at the University in Leicester, England, who also contributed an electronic board to the project, and colleagues from the IRFU institute in Saclay, France. The latter have contributed the analog readout chip NECTAr, which was designed for application in the Cherenkov telescope array (CTA), and found its first technical application in the new H.E.S.S. camera.
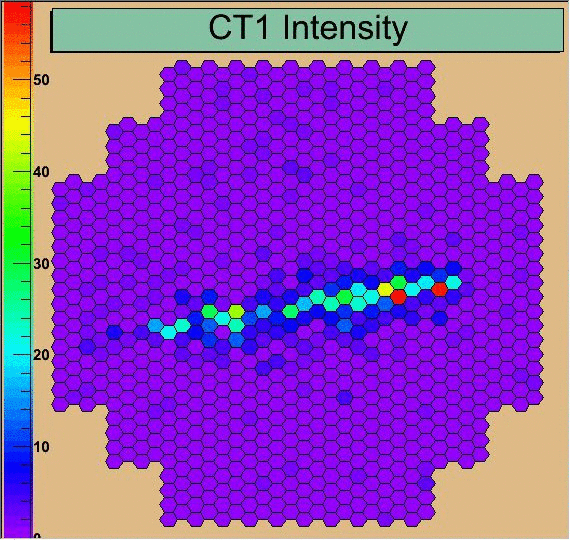
Some of the first gamma-ray images recorded by the new camera.
(credit: The H.E.S.S. collaboration)
Press release: DESY
01/07/2015 - Double Doctoral Degree of KIT and UNSAM
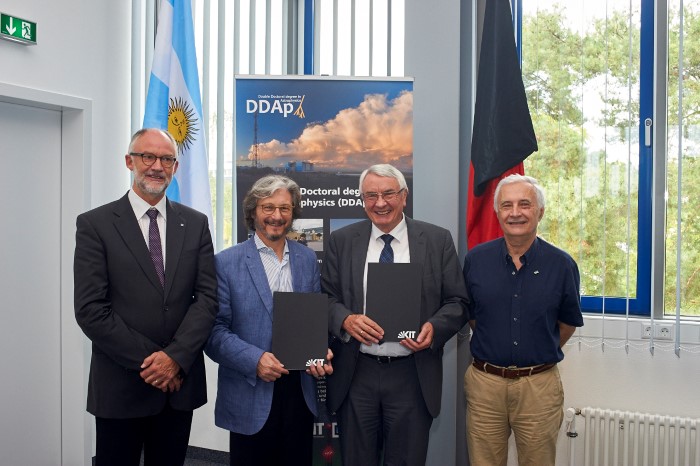
From left to right: Johannes Blümer (spokesperson of KCETA), Carlos Ruta (rector of UNSAM),
Detlef Löhe (vice-President of KIT), Alberto Etchegoyen (director of ITeDA)
(credit: KIT)
On Sept 1st, the Karlsruhe Institute of Technology (KIT) and the Universidad Nacional de San Martin (UNSAM) celebrated their Double Doctoral degree in Astrophysics (DDAp), which started this year. German and Argentinean scientists, academic authorities, professors and students were gathered to officially kick-off the double degree at KIT.
The programm of the day was to present the DDAp to the future graduate students and to highlight the long lasting friendship between UNSAM and KIT. Detlef Löhe, Vice-President of KIT and Carlos Ruta, Rector of UNSAM welcomed the participants and presented their respective university, while Johannes Blümer, spokesperson of the KIT Center Elementary Particle and Astroparticle Physics (KCETA) and Alberto Etchegoyen, director of the Institute of Technology and Astroparticle Detection (ITeDA), Buenos Aires, highlighted the close connection between KIT and UNSAM over the last decades, especially within the Pierre Auger Observatory, and the huge potential of cooperation for the future.
The celebrations continued with an overview about the development of nuclear physics at Forschungszentrum Karlsruhe by Manfred Popp. Then Frank Schröder described the concept and the organisation of the DDAp and Ewa Holt, one of the first DDAp participants, gave her impressions on her first half year in Buenos Aires within the frame of the programme. It was followed by a talk of Bernd Müller-Jacquier (Universität Bayreuth), who described the linguistic problems that could occur when working in intercultural environments. Finally Alan Watson (University of Leeds), Spokesperson Emeritus of the Pierre Auger Observatory, presented a history of research on high-energy particle physics.
The Double Doctoral degree in Astrophysics (DDAp) is jointly offered by the two HAP-partners the Physics Department at the Karlsruhe Institute of Technology (KIT) in Germany and the Institute of Technology in Detection and Astrophysics (ITeDA)
at the Universidad Nacional de San Martín (UNSAM) in Buenos Aires, Argentina. Doctoral researchers participating in the DDAp work on joint research projects in astroparticle physics, e.g., related to the Pierre Auger Observatory ,
and spend at least one year in the other country. The doctoral researchers have to follow the rules of both universities and a specific cooperation agreement. The research project is complemented by a lecture program covering astrophysics, astroparticle and particle physics as well as language courses. At the conclusion of a project after typically three years, KIT and UNSAM, will conduct a joint examination and both will issue their doctoral degree.
Over the last years, doctoral researchers already beneficiated of the opportunity to spend part of their doctoral work abroad either at KIT or at UNSAM. These research periods will now cumulate for a double degree. Last May, similar kick-off celebrations already took place at UNSAM. Eight doctoral researches are already participating in the double degree programme.
The DDAp is supported by the German Argentinean University Center (CUAA-DAHZ), BMBF and DAAD in Germany, and Ministerio de Educación and Ministerio de Cienca, Tecnologia e Innovación Productiva in Argentina.
Double Doctoral degree in Astrophysics
Coordinator of the DDAp at KIT is Prof. Blümer.
Questions regarding the DDAp can also be addressed to Dr. Frank Schröder.
Coordinator of the DDAp at ITeDA/UNSAM is Prof. Etchegoyen.
More pictures: indico website of the Kick-Off event
31/07/2015 - CTA Consortium Spokesperson Prof. Werner Hofmann Wins Yodh Prize
|
|
Werner Hofmann, Consortium Spokesperson
of the Cherenkov Telescope Array
(credit: WeAreTheNight.com / Igor Roelofsen)
|
Picture artist of the future CTA array
(credit: IEEEC)
|
Prof. Werner Hofmann, CTA Consortium Spokesperson, was awarded the Yodh Prize "for his outstanding leadership in the field of high-energy gamma-ray astronomy" at the 34th International Cosmic Ray Conference in The Hague, The Netherlands, where CTA Consortium members willcontribute eight talks and 59 posters. The Yodh Prize recognizes a scientist whose research career has had a major impact on the understanding of cosmic rays. It was endowed by Gaurang and Kanwal Yodh to the University of California Irvine Foundation in 1998.
Prof. Hofmann was selected by an international committee of distibguished scientists in the field of cosmic rays and astroparticle physics. The award is administered through the Commission on Astroparticle Physics of the Unternational Uniuon of Pure and Applied Physics (IUPAP) and is presented every two years at the ICRC. In addition to a cash prize, the winner is invited to present a Department of Physics and Astronomy Colloquium at the University of California, Irvine.
Press release: CTA
17/07/2015 - 2015 GNN Dissertation Prize: two winners at DESY
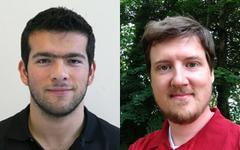
GNN Prize winners Juan Pablo Yáñez (left) und Jakob van Santen
|
A twofold pleasure for the IceCube group at DESY: Juan Pablo Yáñez and Jakob van Santen, both from DESY in Zeuthen, have won two of the three dissertation prizes of the Global Neutrino Network, GNN.
Juan Pablo Yáñez has written his thesis “Measurement of neutrino oscillations in atmospheric neutrinos with the IceCube DeepCore detector” in DESY, as a student of the Humboldt University Berlin. He has analyzed neutrino events taken with IceCube. He could constrain the allowed region for the two parameters which govern the oscillation behavior of neutrinos. That was the first demonstration that a detector in natural ice is able to deliver precision results in the field of neutrino physics. A paper with his results has been published in Phys. Rev. D 91, 072004 (2015) (see also arXiv:1410.7227).
Jakob van Santen has written his thesis not in DESY but as a student of the University of Wisconsin (USA) and could be recruited afterwards as a postdoc for the group in Zeuthen. In his thesis “Neutrino Interactions in IceCube above 1 TeV: Constraints on Atmospheric Charmed-Meson Production and Investigation of the Astrophysical Neutrino Flux with 2 Years of IceCube Data taken 2010-2012” he has analyzed the energy spectrum and angular distribution of neutrinos which have be recorded with IceCube. His work revealed new, important information on the recently discovered astrophysical neutrinos. A paper with his results has been published in Physical Review D 91, 022001 (2105) (see also arXiv:1410.1749).
The Global Neutrino Network was founded on October 15, 2013, with the goal to coordinate construction, operation and data analysis of the large neutrino telescopes in deep water and ice. This year, the GNN Dissertation Prize was awarded for the first time. It acknowledges the work of candidates who have contributed significantly to the success of their project and who have written an outstanding PhD thesis.
Press release: DESY
|
16/07/2015 - Paranal and La Palma sites chosen for final negotiations to host world's largest array of gamma ray telescopes
|
|
|
|
Members of the CTA Resource Board also visited the
telescope prototype in Berlin during their meeting in Zeuthen.
(credit: DESY)
|
Paranal and La Palma have made to final selections to host CTA
(credit: CTA Collaboration)
|
On 15 and 16 July 2015, the Resource Board of the Cherenkov Telescope Array (CTA) decided to enter into detailed contract negotiations for the Northern ans the Southern sites which will host CTA. As for the Northern site the European Southern Observatory (ESO) Paranal grounds in Chile and as for the Southern site the Instituto de Astrofisica de Canarias (IAC), Roque de los Muchachos Observatory in La Palma, Spain.
The Board, composed of representatives of ministries and funding agencies from Austria, Brazil, the Czech Republic, France, Germany, Italy, Namibia, the Netherlands, Japan, Poland, South Africa, Spain, Switzerland and the UK, decided after months of negotiations and careful consideration of extensive studies of the environmental conditions, simulations of the science performance and assessments of construction and operation costs to start contract negotiations with ESO and Spain. The Namibian and Mexican sites will be kept as viable alternatives.
"All sites considered in this final round were of high quality, and the enthusiasm and strong support of CTA by the site proponents made this a very difficult decision for the Resource Board,” said CTA Spokesperson Werner Hofmann
(MPI Heidelberg). "This decision is an important step towards realization of CTA," said Chair of the CTA Resource Board Beatrix Vierkorn-Rudolph (Bundesministerium für Bildung und Forschung).
Vice-Chair Giampaolo Vettolani added, "We hope our supporters and scientists from Namibia and Mexico will continue to contribute scientifically and technically to CTA, driven by the common interest to build the best possible instrument for the entire community." In order to optimize the coverage of the night sky, the CTA Observatory will consist of about 100 telescopes on the southern site and about 20 telescopes on the northern site.
"This is a significant step towards realizing CTA as the most advanced instrument on the planet for very high-energy gamma-ray astronomy and towards making CTA's full power available to the science community early in the next decade," said CTA Co-Spokesperson Rene Ong.
Paranal, Chile (credit: ESO)
The southern site is less than 10 km southeast of ESO’s existing Paranal Observatory in the Atacama Desert, which is considered one of the driest and most isolated regions on earth – an astronomical paradise. In addition to the ideal conditions for year-round observation, collaboration with ESO offers CTA the opportunity to take advantage of existing infrastructure (roads, accommodation, water, electricity, etc.) and access to established facilities and processes for the construction and operation of the observatory.
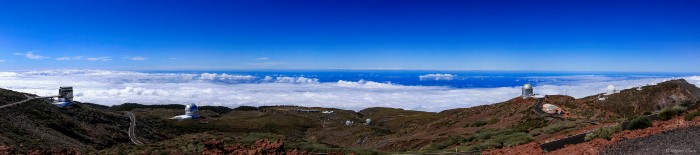
La Palma, Spain (credit: Miguel Claro)
The northern site is located on the existing site of the Instituto Astrofisica de Canarias Observatorio del Roque de los Muchachos on the island of La Palma, the fifth largest island in the Canary Islands. At 2,200 m altitude and nestled on a plateau below the rim of an extinct volcanic crater, the site currently hosts the two Major Atmospheric Gamma Imaging Cherenkov Telescopes (MAGIC) telescopes. This location offers an ideal atmosphere that is mostly free and clear of pollution and turbulence for year-round observation.
Currently in its pre-construction phase, determining the array sites is a critical factor in the CTA construction project. “The selection of array sites is crucial to the progress of CTA and has many implications for the project plans and designs,” said CTA Project Manager Christopher Townsley.
CTA is an initiative to build the world’s largest and most sensitive high-energy gamma-ray observatory. Over 1,000 scientists and engineers from 5 continents, 31 countries and over 170 research institutes participate in the CTA project. CTA will serve as an open observatory to a wide astrophysics community and provide a deep insight into the non-thermal, high-energy universe. The CTA observatory will detect high-energy radiation with unprecedented accuracy and approximately 10 times the sensitivity of current instruments, providing novel insights into some of the most extreme and violent events in the universe.
Press release: CTA [PDF]
30/04/2015 - Prestigious award to IceCube pioneer
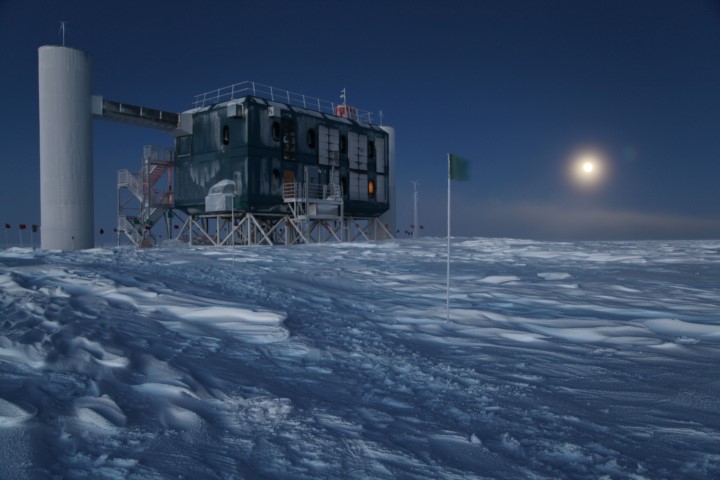
Francis Halzen, principal investigator of the IceCube - South Pole Neutrino Observatory
The European Physical Society (EPS) awards its 2015 Giuseppe and Vanna Cocconi Prize for an outstanding contribution to Particle Astrophysics and Cosmology to IceCube
principal investigator Francis Halzen (University of Wisconsin, Madison). The prestigious price acknowledges "his visionary and leading role in the detection of very high-energy extraterrestrial neutrinos, opening a new observational window on the Universe".
"I feel very honored to receive this prize," says Halzen. "But I understand it firstly as recognition of a great collective effort of the whole IceCube collaboration." This is the second important international appreciation following IceCube's 2013 discovery of cosmic neutrinos, the first being the award "Breakthrough of the Year 2013" by the journal Physics World.
The IceCube physicists from Germany share Halzen's way since more than 20 years and hosted him for frequent working stays in Zeuthen and elsewhere. HAP members played a significant role in breaking the wall to the high-energy neutrino Universe.
Christian Spiering, DESY
30/04/2015 - Latest result from neutrino observatory IceCube opens up new possibilities for particle physics

The IceCube laboratory at the Scott Amundsen South Pole station
hosts the computers collecting the detector data.
(picture: Felipe Pedreros. IceCube/NSF)
The South Pole observatory IceCube has recorded evidence that elusive elementary particles called neutrinos changing their identity as they travel through the Earth and its atmosphere. The observation of these neutrino oscillations, first announced in 1998 by the Super Kamiokande experiment in Japan, opens up new possibilities for particle physics with the Antarctic telescope that was originally designed to detect neutrinos from faraway sources in the cosmos."We are very pleased that the IceCube detector with its DeepCore array can be used to observe neutrino oscillations with high precision," says Olga Botner, Spokesperson of the IceCube experiment. "DeepCore was designed on the initiative of Per Olof Hulth who sadly passed away recently, to significantly lower IceCube's energy threshold. The results show that IceCube can contribute to nailing down the oscillation parameters and motivate us to pursue our plans for an IceCube upgrade called PINGU to measure neutrino properties."
"IceCube records over one hundred thousand atmospheric neutrinos every year, most of them muon neutrinos produced by the interaction of fast cosmic particles with the atmosphere," says Rolf Nahnhauer, leading scientist at DESY. The subdetector DeepCore allows for detecting neutrinos with energies down to 10 giga-electronvolts (GeV). "According to our understanding of neutrino oscillations, IceCube should see fewer muon neutrinos at energies around 25 GeV that reach IceCube after crossing the entire Earth," explains Rolf Nahnhauer. "The reason for these missing muon neutrinos is that they oscillate into other types." IceCube researchers selected Northern Hemisphere muon neutrino candidates with energies between a few GeV and around 50 GeV from data taken between May 2011 and April 2014. About 5 200 events were found, much below the 7 000 expected in the non-oscillations scenario.
Neutrinos remain the most mysterious of the known elementary particles. Postulated by Austrian physicist Wolfgang Pauli in 1930, it took 25 years for their experimental detection. "Neutrinos are elusive," says Olga Botner, " and can travel through an enormous amount of material, even the whole Earth, without interacting." Nevertheless, physicists have built more and more sophisticated instruments to reveal the mysteries of this very light particles. One of the surprising results was that the three different types of neutrinos, electron, muon and tau neutrinos, can change their identity, transforming from one type of neutrino to another. This phenomenon is known as neutrino oscillation. "Neutrino oscillations are only possible if neutrinos have a mass," explains Nahnhauer. "On the other hand, massive neutrinos are not explained within the otherwise so successful Standard Model of particle physics." The strength of the oscillation and the distances over which it develops depend on two parameters: the so-called mixing angle and the mass difference. The values of these parameters have been constrained by precise measurements of neutrinos from the sun, the atmosphere, nuclear reactors, and particle accelerators.
The IceCube neutrino observatory at the South Pole has already demonstrated that it is a powerful tool to explore the universe by neutrinos, using the Antarctic ice sheet as its detection material. An array of more than 5 000 optical sensors distributed in a cubic kilometer of the ice records the very rare collisions of neutrinos. And less than two years ago, IceCube physicists announced the discovery of the first high-energy neutrinos from the cosmos, acknowledged as "Breakthrough of the Year 2013" by the journal Physics World.
Artistic rendering of IceCube DOMs
(digital optical modules) in the Antarctic ice.
(picture: Jamie Yang, IceCube Collaboration)
Now IceCube has proven that it can also deliver top particle physics results. The new measurement by the IceCube collaboration resulting in significantly improved constraints on the neutrino oscillation parameters has been accepted for publication by the scientific journal Physical Review D.
Three years of IceCube data yielded a similar precision to that reached from about 15 years of Super-Kamiokande data. In contrast to the purified water in Super-Kamiokande’s 50-kiloton vessel, IceCube uses a natural target material, the glacier ice at the South Pole. IceCube’s 500 times larger observation volume produces larger event statistics in shorter times. "Both Super-Kamiokande and IceCube use the same ‘beam‘ which is atmospheric neutrinos, but at different energies. And we reach similar precision of the measurable oscillation parameters," says Juan Pablo Yanez, postdoctoral researcher at DESY, who is the corresponding author of the paper. "The results now derived from IceCube data show errors still larger than, but already comparable to the most precise neutrino beam experiments MINOS and T2K. But as IceCube keeps taking data and improving the analyses we are hopeful to catch up soon." adds Yanez.
Currently the scientists are planning an upgrade of the IceCube detector called PINGU (Precision IceCube Next Generation Upgrade). A much higher density of optical modules in the whole central region will improve the sensitivity to several fundamental questions associated with neutrinos.
"In particular we want to measure the so called neutrino mass hierarchy – whether there are two heavier neutrinos and one light one, or whether it is the other way around." explains Rolf Nahnhauer. "This is important to understand how neutrinos obtain masses, but also has significant relevance on how the cosmos evolves. The current results provide an important experimental confirmation that our concepts work."
Press release: DESY
Publication: Measurement of the Atmospheric νe Spectrum with IceCube, IceCube Collaboration, submitted to Physical Review D (arXiv:1504.03753)
More reading: CERN Courier, IceCube website
29/04/2015 - Gamma-ray bursts as cosmic particle accelerators
|
|
|
|
Artist's view of a gamma-ray burst.
(credit: NASA)
|
The plasma is ejected in shells at different speeds. When the shells collide, particles are accelerated.
(illustration: Mauricio Bustamante/DESY)
|
A new study provides detailed insight into the most powerful explosions in the universe: gamma-ray bursts. The simulation explains the modes of particle acceleration in these rare events better than previous models and can explain conflicting astrophysical observations. Scientists from DESY and two US universites present their work in the journal Nature Communications.
Gamma-ray bursts happen when extremely massive stars go supernova. These explosions can be seen nearly across the whole visible universe, up to several billion lightyears. The giant stars' strong magnetic fields channel most of the explosion's energy into two powerful jets of electrically charged gas (plasma), one at each magnetic pole. These plasma jets are powerful natural particle accelerators.
Scientists expect the plasma jets to be a significant source of cosmic rays, high-energy subatomic particles (mostly protons) that constantly pepper Earth's atmosphere from space. These particles can have up to ten million times the energy of the protons in the Large Hadron Collider (LHC), currently the most powerful particle accelerator on Earth at the European particle physics laboratory CERN.
But if gamma-ray bursts are a significant source of cosmic rays, scientists expect them for physics reasons to also shed a large number of light elementary particles called neutrinos. The IceCube Neutrino Observatory at the South Pole, in which DESY is the main European partner, is looking for exactly those high-energy cosmic neutrinos. However, none have been detected so far from gamma-ray bursts. This means that at least ten times fewer neutrinos reach us from gamma-ray bursts than were expected. "This throws up new questions for theory," says DESY scientist Walter Winter, a co-author of the new study. "Perhaps, our concept of gamma-ray bursts was too simple."
Neutrinos are mainly generated at lower distance from the source, cosmic rays
at medium distance and gamma-rays at greater distance.
(illustration Mauricio Bustamante/DESY)
Existing models of these powerful explosions assumed that cosmic rays, neutrinos and gamma-rays all come from the same region within the plasma jets. The team of theoretical astroparticle physicists, including Winter from DESY, Mauricio Bustamante from Ohio State University and Philipp Baerwald and Kohta Murase from Pennsylvania State University, has now developed a more dynamic model of gamma-ray bursts. According to this model, the plasma is ejected in the form of shells at different speed. In considerable distance from the source, these shells collide, thereby accelerating particles.
This approach can not only explain the observed strong variations in the light curves of gamma-ray bursts. A consequence of this model is also that neutrinos, cosmic rays and gamma-rays must be produced in completely different regions of the jets. This can explain, why the expected flux of neutrinos could not be found. “We expect that the next generation of neutrino telescopes, such as IceCube-Gen-2, will be sensitive to this minimal flux that we're predicting”, says Bustamante. In contrast to earlier models, this estimate is more robust and does only weakly depend on the characteristics of individual gamma-ray bursts.
Press release: DESY
Publication: Neutrino and cosmic-ray emission from multiple internal shocks in gamma-ray bursts, Mauricio Bustamante, Philipp Baerwald, Kohta Murase & Walter Winter, Nature Communications 6, Article number: 6783, 2015 (arXiv:1409.2874)
22/04/2015 - EU Millions Grant for Neutrinos Research

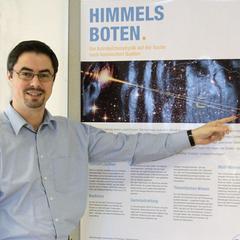
Walter Winter receives an ERC Grant
for his projekt NeuCos.
|
The European Research Council (ERC) is funding a research project of DESY scientist Walter Winter with a so-called ERC Consolidator Grant. Walter Winter of Zeuthen is getting support for five years with 1.75 million euros for his project "NeuCos – Neutrinos and the origin of the cosmic rays". The well endowed ERC Consolidator Grant is awarded to excellent highly talented scientists to promote their independent and separate research activities in the framework of the EU program "Horizon 2020".
Walter Winter, who also teaches at the Humboldt University of Berlin (HU Berlin), will investigate cosmic rays in details with his project. More than 100 years after their discovery, their sources is still a mystery. It has to be gigantic particle accelerators, whose exact origin, especially at the highest energies, is yet unknown. Possible candidates to accelerate these cosmic particles are gamma-ray bursts or active galactic nuclei.
|
Another mystery of cosmic rays is their chemical composition: conclusions from the Pierre Auger Observatory indicate heavier atomic nuclei at the highest energies – a result that raises more questions. At the same time observations of the highest-energy neutrinos with the IceCube Neutrino Observatory revolutionized high-energy astrophysics in 2013. For the first time in addition to photons and cosmic rays, another piece of the puzzle about the nature of cosmic accelerators was found.
One goal of the research project "Neutrinos and the origin of the cosmic rays" is to seek for a common basis for these different observations. Therefore Winter plans to establish a multidisciplinary theory working group with expertise in astrophysics and particle physics. They will develop theoretical models for the sources of heavier nuclei
to extract new indications of the origin of cosmic rays from the observational data of the various cosmic messengers. A further aim is to investigate the particle physics properties of neutrinos with the help of future neutrino telescopes.
Press release: DESY
23/03/2015 - Two new associated partners for HAP

The Helmholtz Alliance for Astroparticle Physics welcomes with great pleasure two new associated partners from Amsterdam, Netherlands:
- the GRavitation and AstroParticle Physics in Amsterdam (GRAPPA) and
- the National Institute for Subatomic Physics (Nikhef).
GRAPPA is a new center of excellence of the University of Amsterdam. It consists of faculty member and outstanding scientists from the Institute for High Energy Physics (IHEF), the 'Anton Pannekoek' Institute (API) and the Institute for Theoretical Physics Amsterdam (ITFA).
The aim of the new center is to bring together theoretical physicists, astronomers and particle physicists, in order to answer some of the most profound questions in Particle Astrophysics and Cosmology.
Nikhef is a partnership between the Foundation for Fundamental Research on Matter (FOM) and four universities: Radboud University Nijmegen, University of Amsterdam, Utrecht University and VU University Amsterdam. The mission of Nikhef is to study the interactions and structure of all elementary particles and fields at the smallest distance scale and the highest attainable energy.
Two complementary approaches are followed: Accelerator-based particle physics and Astroparticle physics.
Nikhef coordinates and leads the Dutch experimental activities in these fields. The research at Nikhef relies on the development of innovative technologies.
23/03/2015 - HAP Annual Member Board Meeting
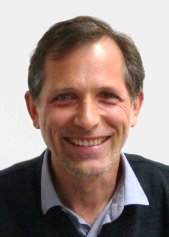
Bianca Keilhauer (KIT) and Josef Jochum (Uni Tübingen) were respectively
elected and reelected Scientific Coordinator and Spokespersons of HAP.
On March 23rd, the Helmholtz Alliance for Astroparticle Physics held its annual HAP Member Board meeting. This meeting was devoted to many elections as designated after the midterm evaluation. Results of the election went as follow:
- Scientific Coordinators, Spokespersons
- Bianca Keilhauer (KIT) and Josef Jochum (Uni Tübingen)
- Administrative Coordinator and Deputy
- Andreas Haungs (KIT) and Marie-Christine Kauffmann (KIT)
- Coordinator Topic 1 - Astroparticle Theory and Deputy
- Günter Sigl (Uni Hamburg) and Karl Mannheim (Uni Würzburg)
- Coordinator Topic 2 - Non-Thermal Universe and Deputy
- Johannes Knapp (DESY) and Daniel Kuempel (RWTH Aachen)
- Coordinator Topic 3 - Dark Universe and Deputy
- Klaus Eitel (KIT) and Gernot Maier (DESY)
- Coordinator Topic 4 - Advanced Technologies and Deputy
- Matthias Kleifges (KIT) and Sebastian Böser (Uni Mainz)
- Coordinator Astronomy and Astrophysics and Deputy
- Martin Pohl (Uni Potsdam) and Markus Ackermann (DESY)
- Coordinator Astronomy and Astrophysics and Deputy
- Martin Pohl (Uni Potsdam) and Markus Ackermann (DESY)
- Coordinator LHC Physics and Deputy
- Thomas Lohse (HU Berlin) and Lutz Köpke (Uni Mainz)
- Coordinator Equal Opportunities
- Gisela Anton (Uni Erlangen) and Julian Rautenberg (Uni Wuppertal)
- Chair of the Member Board
- Christian Stegmann (DESY)
The 3 representatives of the Member Board to be in the Executive Board (EB) will be elected shortly. The EB will also now welcome an ex-Officio KAT representative. All details of the new member board can be found here and the revised HAP bylaws can be found on the HAP Sharepoint.
Two new Senior Fellows were also elected: Claus Grupen (Uni Siegen) and Hinrich Meyer (Uni Wuppertal). HAP also welcomed two new members with their groups: Thomas Bretz (RWTH Aachen) and Stefan Funk (FAU
Erlangen-Nürnberg).
Furthermore, it was announced that two new Associated
Partners joined HAP: the Gravitation AstroParticle Physics Amsterdam (GRAPPA) and
the National Institute for Subatomic Physics (NIKHEF), both from Amsterdam, Netherlands.
22/01/2015 - Gamma-ray telescope H.E.S.S. discovers three most extremely luminous gamma-ray sources in Milky Way’s satellite galaxy
The H.E.S.S. mirror telescopes in Namibia, recently complemented with a 28-metre mirror.
(Picture: © Christian Föhr/ H.E.S.S. Collaboration, 2012)
Once again, the High Energy Stereoscopic System, H.E.S.S., has demonstrated its discovery potential. In the Large Magellanic Cloud, it discovered most luminous very high-energy gamma-ray sources: three objects of different type, namely the pulsar driving wind nebula of the most powerful observed pulsar ever, a most powerful supernova remnant, and a so-called superbubble – a bowl-shaped formation 270 light years in diameter blown by multiple supernovae and stars. This is the first time that a population of stellar-type gamma-ray sources has been detected in an external galaxy, at these highest gamma-ray energies. The superbubble represents the first documented kind of a new source class in very high-energy gamma-rays. The H.E.S.S. Collaboration presents its results in the latest edition of the science journal "Science".
“For a total of 210 hours of measuring time with the H.E.S.S. telescopes we could resolve three fundamentally different sources of high-energy gamma-rays”, said H.E.S.S. spokesperson Prof. Dr Christian Stegmann (DESY). “This shows the huge discovery potential behind the use of combined reflecting telescopes.
Cosmic particle accelerators, mostly supernova remnants and pulsar wind nebulas, i.e. end products of massive stars, are sources of intensive high-energy gamma-rays. They accelerate charged particles to extremely high velocities. When these particles hit the gas or light that surrounds them, gamma-rays are emitted. Most energetic gamma-rays from the cosmos can be observed with extremely sensitive measuring instruments from the surface of the Earth. Entering into the atmosphere they cause cascades of charged secondary particles, so-called particle showers. These emit extreme short, bluish light flashes (Cherenkov light) , which can be detected with big mirror telescopes and rapid light sensors.
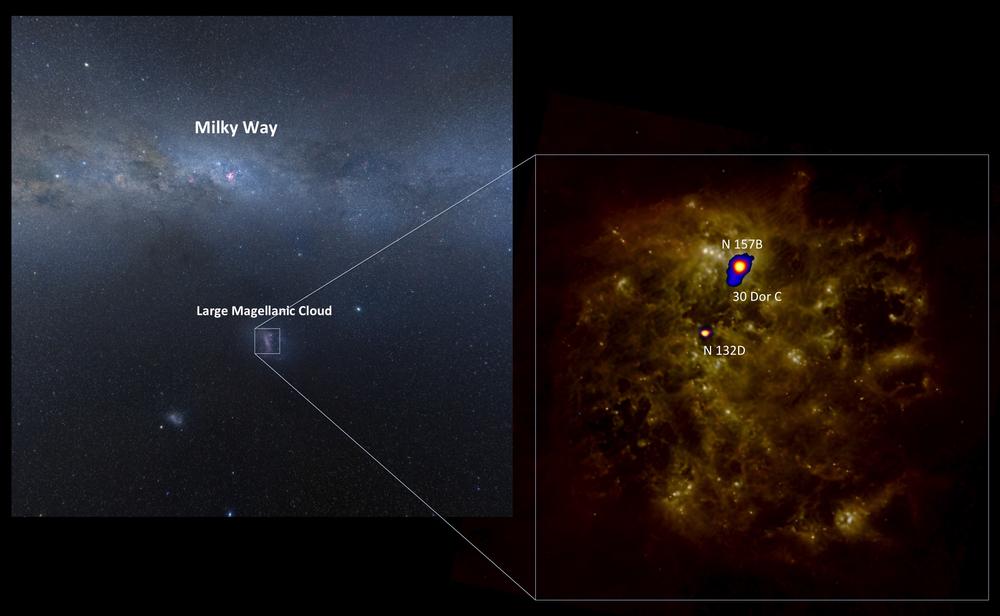
Optical image of the Milky Way and an infrared zoom into the Large Magellanic Cloudwith superimposed H.E.S.S. sky maps.
(Milky Way image: © H.E.S.S. Collaboration, optical: SkyView, A. Mellinger; LMC image © H.E.S.S. Collaboration,
infrared: M. Braun et al. (1997), http://dirty.as.arizona.edu/~kgordon/research/mc/mc.html))
The Large Magellanic Cloud (LMC
)
is a dwarf satellite galaxy of our Milky Way, located about 170 000 light years away. New stars are constantly being formed in it and it harbours numerous massive stellar clusters. The rate by which the new massive stars are formed and explode as supernovae at the end of their lifetime is, relative to its stellar mass, five times higher in the LMC than in the Milky Way. That is one of the reasons why the scientists of the H.E.S.S. Collaboration study these cosmic objects intensively. They examine their most energetic gamma-rays in order to understand the mechanism of particle acceleration in young supernova explosions.
For a total of 210 hours astroparticle physicists aligned the H.E.S.S. telescope on the largest star-forming region within the LMC: the Tarantula Nebula. For the first time they could map several sources of highest energetic gamma-rays in a galaxy outside the Milky Way at the same time and spatially resolved; on top of that they studied three different and extreme high-energetic objects.
The so-called superbubble "30 Dor C" is the largest known X-ray-emitting shell and appears to have been created by several supernovae and strong stellar winds. Until now, it was unclear if superbubbles produce cosmic rays in addition to supernovae remnants. The H.E.S.S. results demonstrate that the bubble is a source, and filled by highly energetic particles. This means that 30 Dor C represents the first proven kind of a new class in very high-energy gamma-ray sources.
The pulsar driving wind nebula observed with the H.E.S.S. telescopes bears the number N 157B. Pulsars are highly magnetised, fast rotating neutron stars which emit a wind of ultra-relativistic particles forming a nebula. The best known example is the Crab Nebula, one of the brightest sources in the high-energy gamma-ray sky. The pulsar of the nebular N 157B in many respects is a twin of the very strong Crab pulsar in our Galaxy. However, N 157B is ten orders of magnitude brighter than the Crab Nebular in the very high-energy gamma-rays. The reasons for this is the lower magnetic field in N 157B and the intense starlight from neighbouring star-forming regions, which both promote the generation of high-energy gamma-rays.
The supernova remnant N 132D is already known as a bright object in the radio and infrared bands. The recent H.E.S.S. measurements show that this object appears to be also one of the oldest – and strongest – supernova remnants glowing in very high-energy gamma-ray light. It is between 2500 and 6000 years old and still outshines the strongest supernova remnant in the Milky Way, although models predict that the expansion of the supernova should be so slow that it is no longer an efficient particle accelerator. “The results confirm the assumption from earlier observations with H.E.S.S. that supernova remnants can be much more luminescent that thought before,” says DESY researcher Stefan Ohm, who made significant contributions to the interpretation and modeling of the LMC data. For measuring such close neighbouring objects scientist have significantly improved the methods for the accuracy of the resolution of the direction where the gamma-rays come from. Future planned experiments in astroparticle physics will benefit. “Just imagine what we could achieve with the more sensitive Cherenkov Telescope Array (CTA) in a few years!” says Ohm.
An electronic upgrade presently developed in Zeuthen will boost the performance of the H.E.S.S. telescope system from 2016 on. For the further future the scientists of the Cherenkov Telescope Array (CTA) plan that as of 2020 the LMC shall provide more sensitive and higher resolving gamma-light images. This galaxy, as the most important object of observation, is high up on the agenda of CTA scientists.
H.E.S.S. - The High Energy Stereoscopic System
The
H.E.S.S. collaboration teams up scientists from Germany, France, Great Britain, Namibia, South Africa, Ireland, Armenia, Poland, Australia, Austria, Holland and Sweden, supported by their respective funding agencies and institutions.
The H.E.S.S. mirror telescopes are situated in Namibia in the southwest of Africa. This system, consisting of four 13-metre diameter telescopes was recently complemented with the 28-metre H.E.S.S. II telescope and is one of the most sensitive detectors of very high-energy gamma rays. When absorbed in the Earth’s atmosphere they create a short-lived shower of particles. The H.E.S.S. telescopes detect the faint bluish flashes which these particle showers emit (named Cherenkov-Light, lasting a few billionths of a second), collecting the light with big mirrors which reflect it onto extremely sensitive cameras. Each image gives the position on the sky of a single gamma-ray photon, and the amount of light collected corresponds to its energy. Building up the images photon by photon allows H.E.S.S. to create maps of astronomical objects as they appear in gamma rays.
The H.E.S.S. telescopes have been operating since late 2002; in September 2012 H.E.S.S. celebrated the first decade of operation, within which the telescopes had recorded 9415 hours of observation, and detected 6361 million air shower events. H.E.S.S. has discovered the majority of the about 150 known cosmic objects emitting highest-energy gamma-rays. In 2006 the H.E.S.S. team was awarded the Descartes Prize of the European Commission and in 2010 the Rossi Prize of the American Astronomical Society. In 2009 a study listed H.E.S.S. among the top 10 observatories worldwide.
|
Press release: DESY
Publication: The exceptionally powerful TeV gamma-ray emitters in the Large Magellanic Cloud, The H.E.S.S. Collaboration, Science, 2015, 347, issue 6220, p. 406 (arXiv:1501.06578)
Enregistrer
16/01/15 - New Chair and General Secretary for APPEC
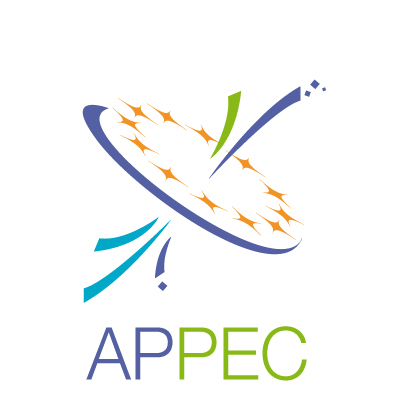
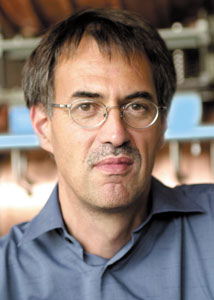
On January 9, 2015 the General Assembly of the Astroparticle Physics European Consortium (APPEC) elected Frank Linde – director of Nikhef from 2004 to 2014 – as its new Chair. He is taking over from Stavros Katsanevas, director of APC, who was chairing APPEC since November 2012. Thomas Berghöfer (DESY) was reappointed as General Secretary. Both appointments will be effective for the coming two years.
About his new appointment Frank Linde said: "Astroparticle physics addresses incredibly tantalizing and fundamental scientific questions. A top priority for me will be to get the large observatories in particular for multi-messenger studies really on track i.e. funded with a realistic spending profile taking into account exploitation costs. From APPEC's perspective we would also benefit from a stronger CERN involvement by learning from CERN’s very professional project review mechanisms as well as by hopefully attracting some of CERN’s resources to astroparticle physics e.g. for R&D activities".
Frank Linde also thanked Stavros Katsanevas for his dedicated work in leading the APPEC consortium and his enthusiastic
impact in forming the astroparticle physics community in Europe during his mandate.
Stavros Katsanevas summarized the first years of the APPEC Consortium taking over from the EU funded
ASPERA ERANET as quite active and formative: "Highlights of our activity have been the effort to
coordinate the community in view of Horizon 2020, the roadmap update and our policy contributions to
the European Particle Physics Roadmap as well as the P5 process in the USA, the preparation of the
triggering events and accompanying measures of the globalisation process in the neutrino sector and the
development of closer relationships with the astrophysics and particle physics communities (ERANET
ASTRONET, ECFA) and the international centres (ESO, CERN). I personally had great pleasure to
collaborate during these last years with the heads of agencies forming APPEC, Janet Seed (STFC) the
Vice-Chair of APPEC, Thomas Berghöfer the General Secretary, Antonio Masiero (INFN) the head of the
APPEC Scientific Advisory Committee, and of course the members of the APPEC functional centres.”
APPEC
Since its launch in June 2012, the APPEC consortium coordinates towards the next generation of
research infrastructures defined in its roadmap, including high energy multi-messenger observatories,
gravitational wave interferometers, experiments to derive neutrino properties and direct dark matter
searches as well as dark energy surveys.
The consortium currently comprises funding agencies from 14 European countries and ESO as an
observer. Negotiations are going on to enlarge the consortium by
partners from Armenia, Austria, Bulgaria, Czech Republic, Estonia, Finland and Portugal.
In the first half of 2015 APPEC is organizing a second global neutrino conference at Fermilab, a
Technology Forum on low-level light detection in Munich, and a cosmology workshop in Florence.
|
Press release: APPEC, Nikhef